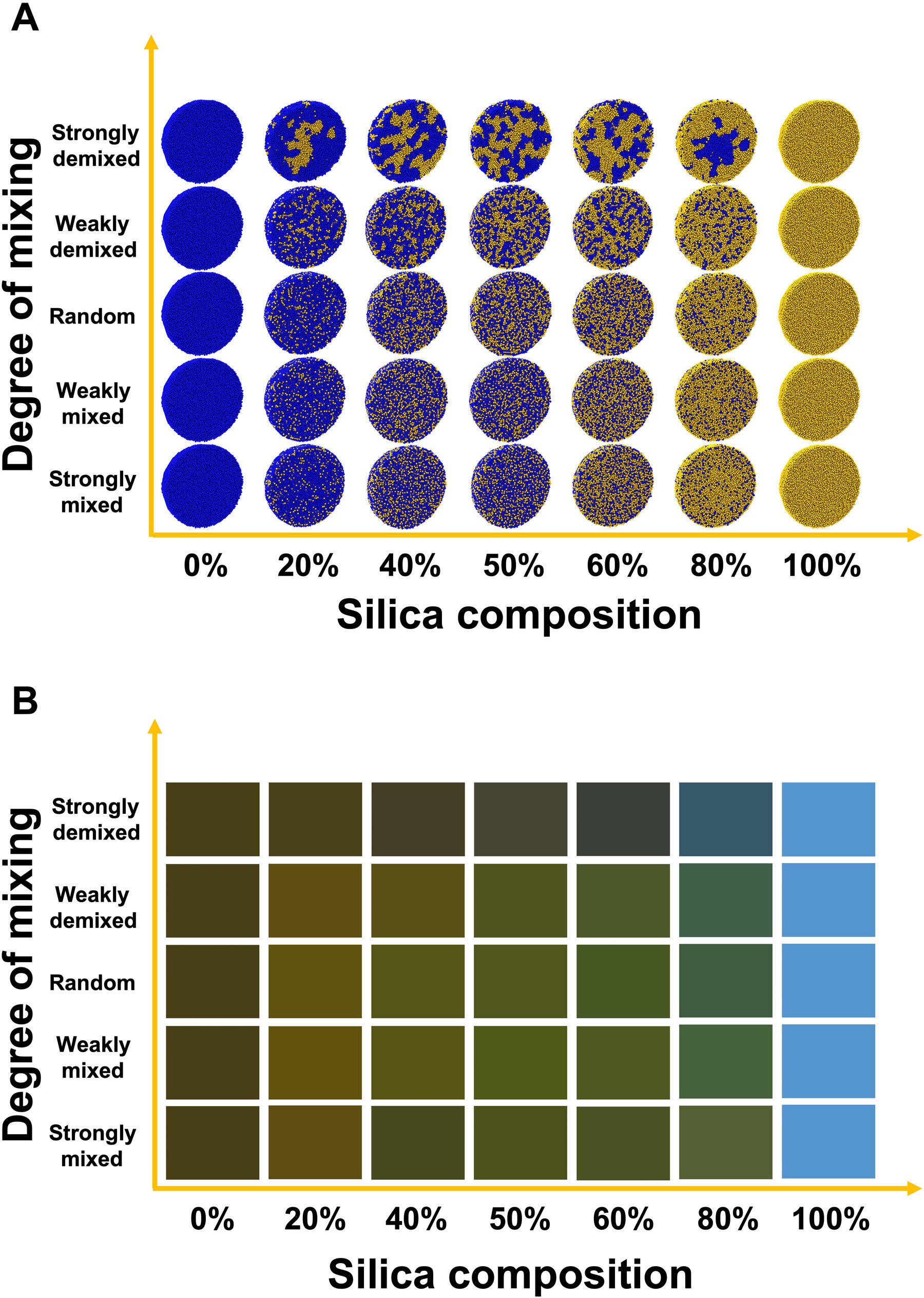
Materials with Bird-Inspired Structural Colors Using Nanoparticle: Materials scientists are often bioinspired, and in a new study, bird-inspired by structural colors exhibited by avian species to form non-iridescent nanoparticle assemblies. Such nanoparticle mixtures varying in particle chemistry and size can affect the color produced to identify structure-color relationships and create designer materials with tailored color.
In a new report on Science Advances, Christian M. Heil, and a research team at several international, multidisciplinary research institutes in the U.S., Belgium, and Germany, showed how to reconstruct the assembled structures via small-angle scattering measurements.
The research team successfully and quantitatively predicted the experimentally observed colors in mixtures with strongly absorbed nanoparticles to demonstrate the influence of a single layer of segregated nanoparticles and produce a color of interest. The versatile computational approaches integrated in this work were useful to engineer synthetic materials with desired colors suited for paints, cosmetics, and food coloring applications.
Synthetic color fabrication and characterization.
The color fabrication of synthetic materials is inspired by diverse arrays of color in nature. They can arise from the spatial organization of nanostructured materials that are resistant to color degradation. Materials with consistent, periodic nanostructures can form iridescent colors, while those with short-range ordering produce non-iridescent colors.
Materials scientists can mimic natural non-iridescent structural colors by self-assembling polymeric nanoparticles via amorphous assemblies of inorganic nanoparticles. They can vary the colors of nanoparticle assemblies by regulating their structure and optical properties to provide increased structural diversity. Scientists can adjust the nanoparticle size ratio between the components and the composition by generating a myriad of diverse structures and structural colors.
Characterizing designer materials
Heil et al. used small-angle scattering and electron microscopy to obtain structural information of the designer materials. They further integrated small-angle neutron and X-ray scattering to provide well-suited methods to examine the nanoscale and bulk structural information. For optical modeling of complex nano-assemblies, they used the finite-difference time-domain (FDTD) method.
The small-angle-scattering experiments generated a scattering experiment profile, which they interpreted with computational reverse-engineering analysis for scattering experiments (CREASE) to generate nanoparticle assemblies.
The researchers combined CREASE-FDTD methods to understand binary nanoparticle assemblies forming supraballs to produce a wide spectrum of colors. The outcomes characterized the optical properties of nanoparticle assemblies and showed the capacity to design colors on demand for wide-ranging applications in paints, cosmetics and food coloring.
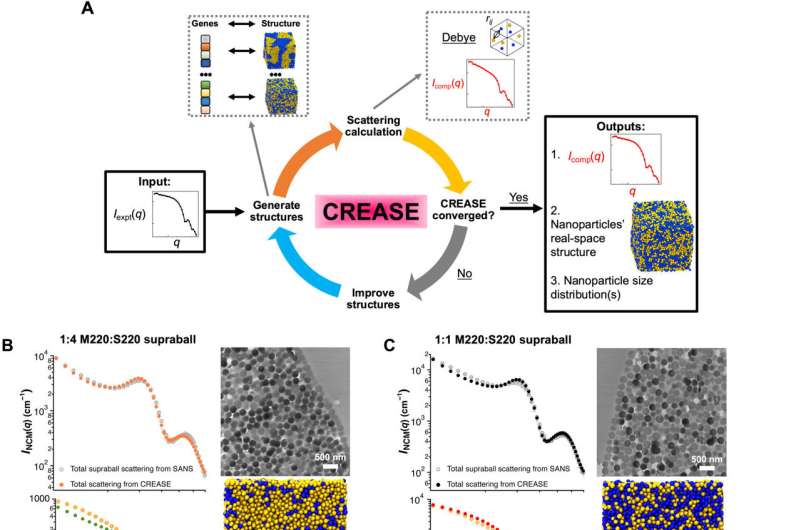
Structural color diversity with binary nanoparticle mixtures
Materials with Bird-Inspired Structural Colors Using Nanoparticle: The materials scientists examined the composition and phase morphology of binary mixtures of absorbing and non-absorbing species of similar size to provide a method to regulate the prediction of structural colors upon which the relative sensitivity of structural color relied. This work produced a rich diversity of colors to show the need to know the relative composition of two nanoparticle types in a binary mixture alongside their phase segregation and the extent of mixing.
To monitor this process, Heil et al. used an experimental and computational approach, which provided information about the internal morphologies of supraballs to predict each reflectance spectra and their resulting color.
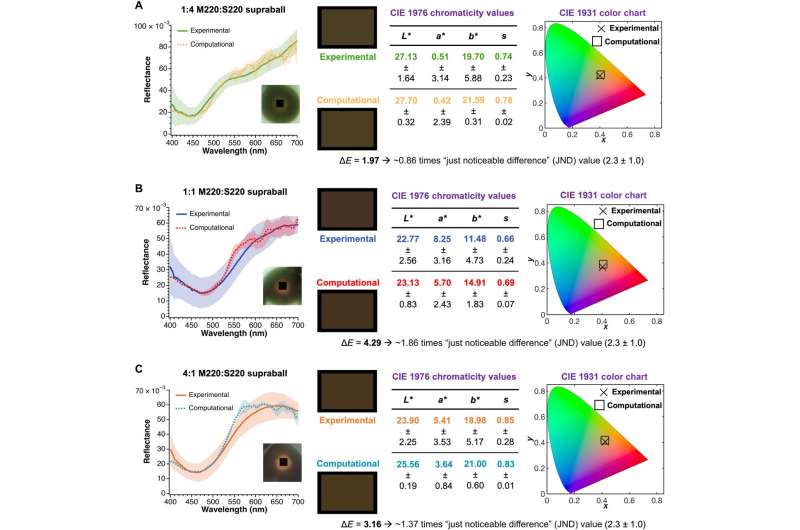
CREASE and small-angle neutron scattering data
Materials with Bird-Inspired Structural Colors Using Nanoparticle: The team conducted small-angle neutron scattering experiments for three different mixtures of nanoparticles and produced suspensions of micron-scale supraballs. They obtained morphological information of the interior of supraballs by isolating the scattering contribution of individual components within the structure to collect total scattering from the nanoparticles of interest.
Using computational reverse-engineering analysis for the scattering experiments, the team analyzed scattering results from multicomponent structures to obtain 3D structural reconstruction and optical simulations of the products.
The outcomes of the structural analysis based on computational reverse-engineering analysis for scattering experiments (CREASE), provided the coordinates of all nanoparticles within supraballs. This information was useful to calculate the scattering of light by employing the finite-difference-time domain method (FDTD).
The combined CREASE-FDTD method represented a close structural match to experimental systems with optical modeling comparisons. Based on the remarkable agreement between these two combined methods, the team modeled optical properties underlying a complex binary mixture of nanoparticles to understand the impact of size dispersity and surface segregation on structural color.
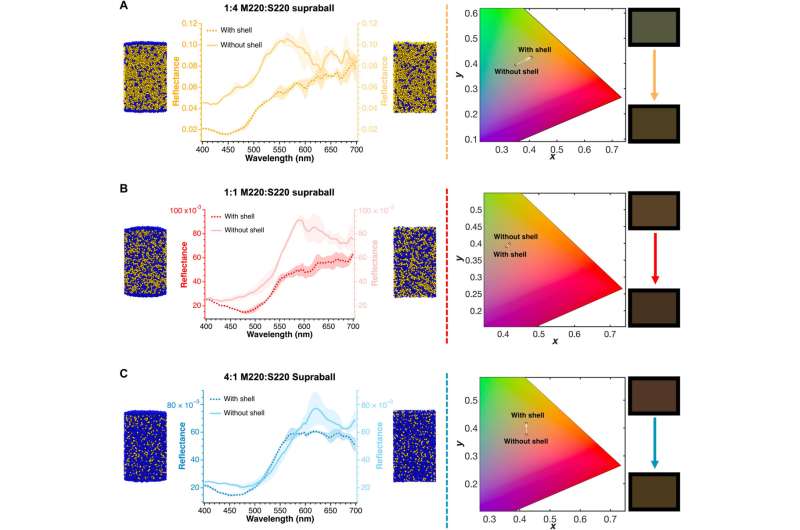
Outlook
In this way, Christian M. Heil and colleagues combined two key methods; computational reverse-engineering analysis for scattering experiment (CREASE) and the finite-difference-time domain method (FDTD) to provide a new platform to model structural colors of nanoparticle-based supra-assemblies. The bird-inspired structural colors formed non-iridescent nanoparticle assemblies, while those with short-range ordering produce non-iridescent colors.
The team used two primary methods to determine the outcomes as a function of size, dispersity, phase morphology and strongly absorbing optical properties of the nanoparticles. This combined method produced reconstructed 3D structures of nanoparticle assemblies with scattering profiles that were a close match to those obtained via small-angle neutron scattering measurements. The combined method is well-suited for multiscale modeling to study optical properties of much larger assemblies of supraballs, such as packed films and pigment dispersions.
This method can design programmable colors for practical applications in coatings, paints and cosmetics to help materials researchers better understand and design complex materials for applications across the electromagnetic spectra, while predicting material properties such as thermal, electrical conductivity and mechanical properties, which depend on varying structural compositions of the designed materials.
Materials with Bird-Inspired Structural Colors Using Nanoparticle: Original Article
Tellurium nanoparticles achieve dielectric properties when exposed to sunlight
Researchers tune material’s color and thermal properties separately
A 200-year-old guide to color, redesigned for the internet age