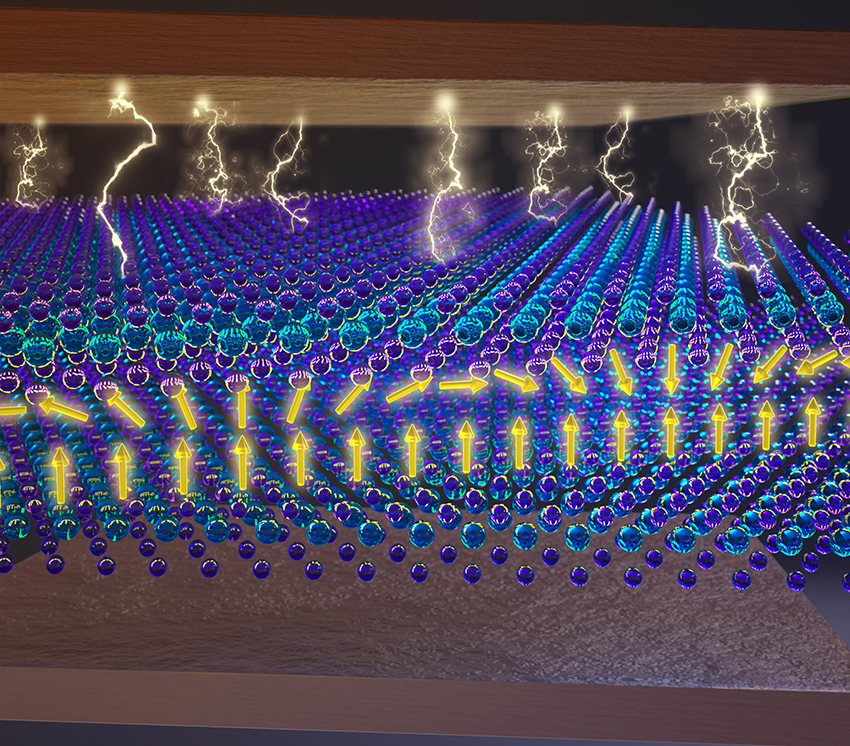
Purdue quantum researchers twist double bilayers of an antiferromagnet to demonstrate tunable moiré magnetism
2D Magnets the Next Giant Leap in Quantum Electronics: Twistronics isn’t a new dance move, exercise equipment, or new music fad. No, it’s much cooler than any of that. It is an exciting new development in quantum physics and material science where van der Waals materials are stacked on top of each other in layers, like sheets of paper in a ream that can easily twist and rotate while remaining flat, and quantum physicists have used these stacks to discover intriguing quantum phenomena.
Adding the concept of quantum spin with twisted double bilayers of an antiferromagnet, it is possible to have tunable moiré magnetism. This suggests a new class of material platform for the next step in twistronics: spintronics. This new science could lead to promising memory and spin-logic devices, opening the world of physics up to a whole new avenue with spintronic applications.
A team of quantum physics and materials researchers at Purdue University has introduced the twist to control the spin degree of freedom, using CrI3, an interlayer-antiferromagnetic-coupled van der Waals (vdW) material, as their medium. They have published their findings, “Electrically tunable moiré magnetism in twisted double bilayers of chromium triiodide,” in Nature Electronics.
“In this study, we fabricated twisted double bilayer CrI3, that is, bilayer plus bilayer with a twist angle between them,” says Dr. Guanghui Cheng, co-lead author of the publication. “We report moiré magnetism with rich magnetic phases and significant tunability by the electrical method.”
The team, mostly from Purdue, has two equal-contributing lead authors: Dr. Guanghui Cheng and Mohammad Mushfiqur Rahman. Cheng was a postdoc in Dr. Yong P. Chen’s group at Purdue University and is now an Assistant Professor in Advanced Institute for Material Research (AIMR, where Chen is also affiliated as a principal investigator) at Tohoku University. Mohammad Mushfiqur Rahman is a PhD student in Dr. Pramey Upadhyaya’s group. Both Chen and Upadhyaya are corresponding authors of this publication and are professors at Purdue University. Chen is the Karl Lark-Horovitz Professor of Physics and Astronomy, a Professor of Electrical and Computer Engineering, and the Director of Purdue Quantum Science and Engineering Institute. Upadhyaya is an Assistant Professor of Electrical and Computer Engineering. Other Purdue-affiliated team members include Andres Llacsahuanga Allcca (PhD student), Dr. Lina Liu (postdoc), and Dr. Lei Fu (postdoc) from Chen’s group, Dr. Avinash Rustagi (postdoc) from Upadhyaya’s group and Dr. Xingtao Liu (former research assistant at Birck Nanotechnology Center).
“We stacked and twisted an antiferromagnet onto itself and voila got a ferromagnet,” says Chen. “This is also a striking example of the recently emerged area of ‘twisted’ or moiré magnetism in twisted 2D materials, where the twisting angle between the two layers gives a powerful tuning knob and changes the material property dramatically.”
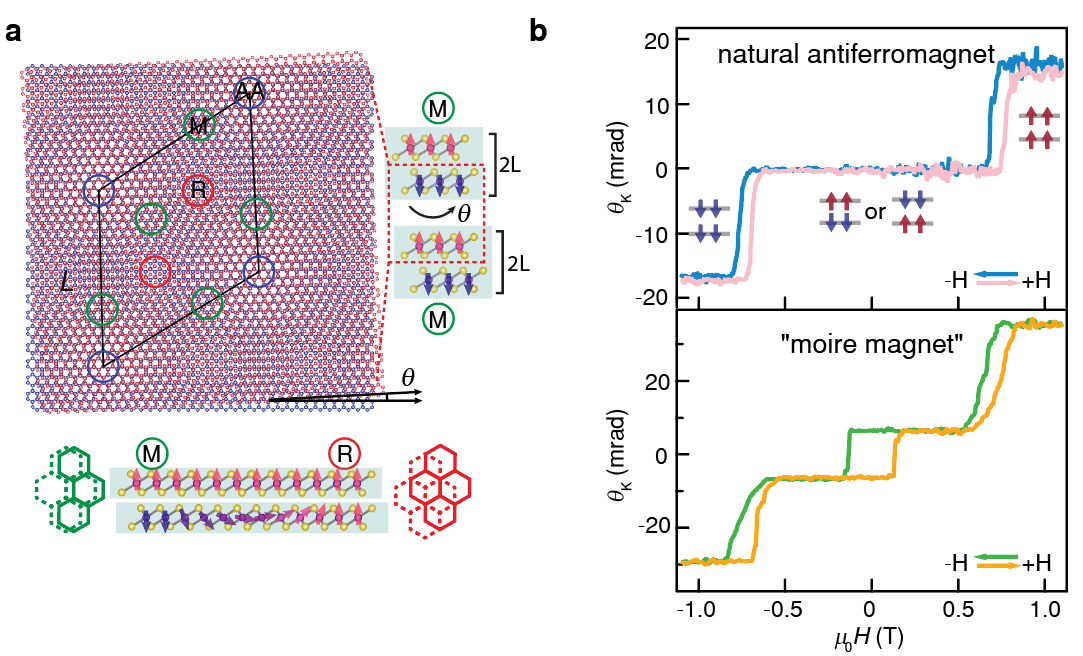
2D Magnets the Next Giant Leap in Quantum Electronics: “To fabricate twisted double bilayer CrI3, we tear up one part of bilayer CrI3, rotate and stack onto the other part, using the so-called tear-and-stack technique,” explains Cheng. “Through magneto-optical Kerr effect (MOKE) measurement, which is a sensitive tool to probe magnetic behavior down to a few atomic layers, we observed the coexistence of ferromagnetic and antiferromagnetic orders, which is the hallmark of moiré magnetism, and further demonstrated voltage-assisted magnetic switching. Such a moiré magnetism is a novel form of magnetism featuring spatially varying ferromagnetic and antiferromagnetic phases, alternating periodically according to the moiré superlattice.”
Twistronics up to this point have mainly focused on modulating electronic properties, such as twisted bilayer graphene. The Purdue team wanted to introduce the twist to spin degree of freedom and chose to use CrI3, an interlayer-antiferromagnetic-coupled vdW material. The result of stacked antiferromagnets twisting onto itself was made possible by having fabricated samples with different twisting angles. In other words, once fabricated, the twist angle of each device becomes fixed, and then MOKE measurements are performed.
Theoretical calculations for this experiment were performed by Upadhyaya and his team. This provided strong support for the observations arrived at by Chen’s team.
“Our theoretical calculations have revealed a rich phase diagram with non-collinear phases of TA-1DW, TA-2DW, TS-2DW, TS-4DW, etc.,” says Upadhyaya.
This research folds into an ongoing research avenue by Chen’s team. This work follows several related recent publications by the team related to novel physics and properties of “2D magnets,” such as “Emergence of electric-field-tunable interfacial ferromagnetism in 2D antiferromagnet heterostructures,” which was recently published in Nature Communications. This research avenue has exciting possibilities in the field of twistronics and spintronics.
“The identified moiré magnet suggests a new class of material platform for spintronics and magnetoelectronics,” says Chen. “The observed voltage-assisted magnetic switching and magnetoelectric effect may lead to promising memory and spin-logic devices. As a novel degree of freedom, the twist can be applicable to the vast range of homo/heterobilayers of vdW magnets, opening the opportunity to pursue new physics as well as spintronic applications.”
This work is partially supported by US Department of Energy (DOE) Office of Science through the Quantum Science Center (QSC, a National Quantum Information Science Research Center) and Department of Defense (DOD) Multidisciplinary University Research Initiatives (MURI) program (FA9550-20-1-0322). Cheng and Chen also received partial support from WPI-AIMR, JSPS KAKENHI Basic Science A (18H03858), New Science (18H04473 and 20H04623), and Tohoku University FRiD program in early stages of the research. Upadhyaya also acknowledges support from the National Science Foundation (NSF) (ECCS-1810494). Bulk CrI3 crystals are provided by the group of Zhiqiang Mao from Pennsylvania State University under the support of the US DOE (DE-SC0019068). Bulk hBN crystals are provided by Kenji Watanabe and Takashi Taniguchi from National Institute for Materials Science in Japan under support from the JSPS KAKENHI (Grant Numbers 20H00354, 21H05233 and 23H02052) and World Premier International Research Center Initiative (WPI), MEXT, Japan.
About the Department of Physics and Astronomy at Purdue University
2D Magnets the Next Giant Leap in Quantum Electronics: Purdue Department of Physics and Astronomy has a rich and long history dating back to 1904. Our faculty and students are exploring nature at all length scales, from the subatomic to the macroscopic and everything in between. With an excellent and diverse community of faculty, postdocs, and students who are pushing new scientific frontiers, we offer a dynamic learning environment, an inclusive research community, and an engaging network of scholars.
Physics and Astronomy is one of the seven departments within the Purdue University College of Science. World-class research is performed in astrophysics, atomic and molecular optics, accelerator mass spectrometry, biophysics, condensed matter physics, quantum information science, particle and nuclear physics. Our state-of-the-art facilities are in the Physics Building, but our researchers also engage in interdisciplinary work at Discovery Park District at Purdue, particularly the Birck Nanotechnology Center and the Bindley Bioscience Center. We also participate in global research including at the Large Hadron Collider at CERN, many national laboratories (such as Argonne National Laboratory, Brookhaven National Laboratory, Fermilab, Oak Ridge National Laboratory, the Stanford Linear Accelerator, etc.), the James Webb Space Telescope, and several observatories around the world.
About the Elmore Family School of Electrical and Computer Engineering
The Elmore Family School of Electrical and Computer Engineering is the largest academic unit at Purdue University and the largest ECE department in the country. Established in 1888, the school offers both undergraduate B.S. degrees as well as M.S. and Ph.D. graduate degrees in Electrical Engineering and Computer Engineering. The school enrolls more than 1,900 undergraduates (sophomores through seniors) and over 1,300 graduate students. Degree programs on the West Lafayette campus are consistently ranked in the top 10 by the US News & World Report. U.S. News also ranks the online master’s program in electrical engineering No. 1 in the nation. Purdue ECE aims to serve and lead the state of Indiana, the nation, and the world-wide profession of electrical and computer engineering, by educating the next generation of engineers, by discovery that advances fundamental knowledge and its applications, and by innovation and engagement that address global challenges of societal impact.
About the Purdue Quantum Science and Engineering Institute (PQSEI)
Located in Discovery Park District, PQSEI fosters the development of practical and impactful aspects of quantum science and focuses on discovering and studying new materials, devices, and basic physical quantum systems that will be suited for integration into tomorrow’s technology. It encourages interdisciplinary collaboration leading to the design and realization of quantum devices with enhanced functionality and performance close to the fundamental limit, aiming to ultimately bring them to a vast community of users. PQSEI faculty work on a broad range of topics in quantum science and engineering, including quantum materials and devices, quantum photonics, atomic molecular and optical physics, quantum chemistry, quantum measurement and control, quantum simulation, and quantum information and computing. Finally, PQSEI works to train the next generation of quantum scientists and engineers in order to meet the growing quantum workforce demands.
About Purdue University
Purdue University is a top public research institution developing practical solutions to today’s toughest challenges. Ranked in each of the last five years as one of the 10 Most Innovative universities in the United States by U.S. News & World Report, Purdue delivers world-changing research and out-of-this-world discovery. Committed to hands-on and online, real-world learning, Purdue offers a transformative education to all. Committed to affordability and accessibility, Purdue has frozen tuition and most fees at 2012-13 levels, enabling more students than ever to graduate debt-free. See how Purdue never stops in the persistent pursuit of the next giant leap at https://stories.purdue.edu.
Written by Cheryl Pierce, Communications Specialist
Contributors: Dr. Guanghui Cheng, Assistant Professor in Advanced Institute for Material Research (AIMR) at Tohoku University and Dr. Yong P. Chen, Karl Lark-Horovitz Professor of Physics and Astronomy, Professor of Electrical and Computer Engineering, and the Director of Purdue Quantum Science and Engineering Institute.
2D Magnets the Next Giant Leap in Quantum Electronics: Original Article
Team finds Wigner crystal—not Mott insulator—in ‘magic-angle’ graphene
Graphene bilayer provides efficient transport and control of spins